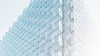
TECHNICAL NOTE
Realization is near! ? What is a quantum sensor?
This time we will explain about ensemble-type magnetic field measurement, which Quemix is particularly focusing on among quantum sensors. A quantum sensor is a device that utilizes quantum properties in materials to sense the environment. Quantum sensors do not require very precise control technology, and are therefore expected to be implemented in society relatively quickly.
The features of quantum sensors include the following.
-
High sensitivity: Can measure magnetic fields, temperature, etc. with extremely high sensitivity
-
Room temperature operation: Unlike other quantum devices, it operates at room temperature and does not require cryogenic cooling.
-
Wide dynamic range: wide range from the minimum value to the maximum value of the measurable signal
Research on this quantum sensor is progressing and reaching the level of practical application. First, we will explain its expected applications.
INTRODUCTION
Expected applications of quantum sensors
In recent years, we have been hearing the term brain tech more and more. This word is a combination of "brain" and "technology" and is used to read medical diagnosis of the brain, thought processes, and even nonverbal emotions and utilize it for product development and services. New industrial fields are attracting attention.
Information is transmitted in the brain as electrical signals, and capturing these electrical signals is the first step in Brain Tech.
In order to measure this electrical signal in the brain, it is impossible to insert electrodes into the brain. When an electric current flows, a magnetic field is generated, and Brain Tech will measure this brain magnetism.
Currently, measuring magnetic fields deep within the brain requires a large, extremely sensitive device called a superconducting quantum interference device (SQUID). However, recently, quantum sensors have been attracting attention as high-sensitivity magnetic field sensors that can replace SQUIDs.
Unlike SQUIDs, quantum sensors do not require cryogenic cooling, making them a promising device for magnetoencephalography measurements. At present, the sensitivity of quantum sensors is still not as high as that of SQUIDs, but laboratory papers have reported performance values that are sufficient for practical use in magnetoencephalography measurements.
APPLICATION
Magnetoencephalography measurement - Braintech -
Other quantum sensor applications
In addition, the following applications are expected within the known range.
-
Monitoring battery capacity on board electric vehicles
-
ocean observation system
-
Investigation of natural phenomena (undersea earthquakes, volcanic activity), etc.
-
Surveillance of suspicious navigational objects
-
Anomaly detection in semiconductor devices
The three essential elements that quantum sensors should fulfill are: Noise and signal are two sides of the same coin.
Quantum sensors must possess the following three essential characteristics, as encapsulated in the earlier statement: "A quantum sensor is a device that utilizes the quantum properties within matter to perform environmental sensing."
-
Capability to Maintain and Measure Controllable Quantum States: Quantum sensors should have the ability to create and measure quantum states that are controllable. This means they can manipulate quantum properties to encode information relevant to the environmental parameter they are sensing.
-
Susceptibility to Perturbations from the External Environment: Quantum states within the sensor should be sensitive to the external environment or the parameter being measured. This sensitivity allows the quantum sensor to change its quantum state in response to variations in the environment, effectively detecting the desired parameter.
-
Existence of Protocols to Eliminate External Interference (Noise) Other Than the Target Parameter: Quantum sensors should have protocols or methods in place to filter out or mitigate interference from sources other than the external environment they are intended to measure. This ensures that the sensor's measurements are as accurate as possible, with minimal interference from unrelated factors.
Interestingly, elements 1 and 3 mentioned above also apply directly to quantum computers. Quantum computers work by creating and controlling quantum states on quantum bits (qubits), performing operations or computations on these qubits, and ultimately measuring the results. In this context, quantum computers are designed to minimize external interference (noise) to ensure the accuracy of their computations.
CONDITIONS
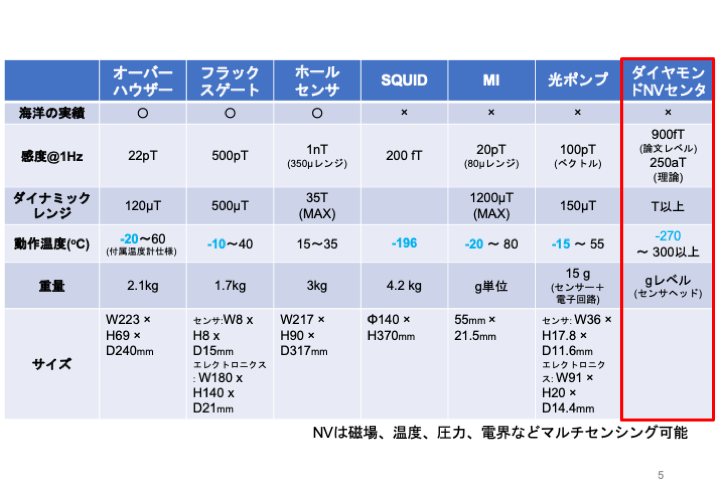
Table 1. Comparison table of quantum sensor (Diamond NV Center) and other sensor devices
Quantum sensor noise reduction protocol development
Quemix focuses on the development of protocols for fulfilling the third requirement mentioned earlier, which is the ability to remove interference (noise) from sources other than the external environment of interest. Quemix leverages its expertise in material calculations to develop such protocols. Although not explicitly explained earlier, in quantum sensors, the duration for which a quantum state can be maintained is referred to as the spin coherence time (T2). Quemix conducts research to extend the spin coherence time, which can lead to increased sensitivity of quantum sensors.
Quemix focuses on the development of protocols for fulfilling the third requirement mentioned earlier, which is the ability to remove interference (noise) from sources other than the external environment of interest. Quemix leverages its expertise in material calculations to develop such protocols. Although not explicitly explained earlier, in quantum sensors, the duration for which a quantum state can be maintained is referred to as the spin coherence time (T2). Quemix conducts research to extend the spin coherence time, which can lead to increased sensitivity of quantum sensors.
It is known that a longer spin coherence time results in higher sensitivity for quantum sensors. Research on extending the spin coherence time is conducted worldwide, but it often relies on experimental trial and error. The spin coherence time is a physical quantity determined by various sources of interference, and as the interference factors decrease, the spin coherence time increases.
Quemix, utilizing its expertise in material calculation techniques, identifies factors that shorten the spin coherence time and works on eliminating them, thereby achieving an extension of the spin coherence time and enhancing the sensitivity of quantum sensors.
In fact, in the fiscal year 2021, as part of the "Defense Ministry's Security Technology Research Promotion System," Quemix is engaged in research and development for quantum sensor applications in underwater sensing, with QST as the prime contractor. In this context, they are working on noise reduction techniques, keeping underwater noise in mind. In real-world operating environments, combining noise reduction techniques tailored to specific environmental conditions can be effective. Quemix is actively involved in the development of the quantum sensor system for such applications.
DEVELOPMENT
Figure 1. Seafloor sensing
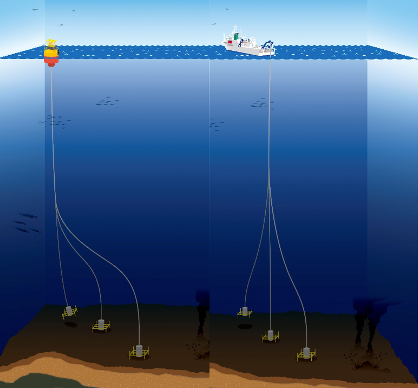
In search of better quantum sensor materials
As I mentioned earlier, research and development is currently underway with diamond NV centers far ahead of other quantum sensor materials. This is because there is a great advantage in controlling the quantum state. However, a longer wavelength of light for quantum state reading would be suitable for long-distance transmission. While having controllability equivalent to that of a diamond NV center, it is possible to read out quantum states at longer wavelengths, and the existence of excellent localized spins with higher readout brightness and longer spin coherence time will revolutionize quantum sensors. We expect further development. By making full use of the material calculation technology that is one of the features of Quemix, we are proceeding with the discovery and demonstration of new quantum sensor materials that are more suitable to replace the diamond NV center.
ADVANCEMENTS
What kind of quantum sensor research is Quemix conducting?
As I have written so far, quantum sensors are a technology that is expected to be realized relatively early among quantum technologies. For Quemix, which aims to bring quantum technology to society as soon as possible, quantum sensor research is one of the important quantum technologies that it is focusing on. Matsushita, who serves as the representative of Quemix, is also involved in quantum sensor research and development as the project chief of the Quantum Materials Theory Project at the National Institute for Quantum Science and Technology (QST). In the quantum sensor field, QST is a research institution with the second largest number of cited papers in the world (first in Japan) and is a major global research center for quantum sensors. Here, we will explain how Quemix is actually involved in the development of quantum sensor technology.
COLLABORATION
Why cutting-edge quantum researchers are paying attention to “defects”
What do you think of when you hear the word 'defect'? In general, 'defect' is a word that carries a very negative connotation. In many contexts, 'defect' is synonymous with 'imperfection' and is often treated as something undesirable. This perception is mirrored in the field of material science, where the ideal crystal (material) without defects is referred to as a perfect crystal, and in the synthesis of new materials, the goal is often to grow or synthesize as 'defect-free' and pristine crystals as possible.
However, unfortunately, in the real world, there are no materials or crystals that are completely devoid of defects. Every material, to a greater or lesser extent, contains defects. What's more, certain types of defects can have a detrimental impact on the performance of devices when they are used. Sometimes, defects can significantly degrade device performance, and at other times, they can greatly compromise device reliability. In some cases, defects can even lead to major accidents; they are, in a sense, 'problematic.'
Therefore, for researchers in device manufacturing, accurately understanding what kinds of defects can cause what types of issues is a crucial area of study.
MATERIAL
Defects - the source of creating novel functionalities within common materials.
Defects - Sources of novel functions in common materials -
n the previous section, we touched upon the negative aspects of defects. Now, let's delve into another aspect of defects, which is their role as a source of "creating novel functionalities within common materials." To illustrate this, let's start with diamond.
Diamond is an insulator composed of carbon atoms and is classified as an ultra-wide bandgap semiconductor with a bandgap of around 5.5 eV. It is highly regarded for its high potential as an ultra-wide bandgap semiconductor and for being the hardest material known. However, when viewed from the perspective of quantum devices, it may initially appear as a rather unremarkable material.
However, diamond becomes a subject of significant interest in the field of quantum devices when nitrogen-vacancy (NV) centers are formed within it. NV centers are defects in diamond where a pair of nitrogen (N) and a vacancy (V) in the carbon lattice are adjacent. The moment these defects are created, diamond instantly garners substantial attention as a quantum sensor material.
Emergence of quantum states at NV centers in diamonds
Diamond NV centers exhibit a significant feature: the appearance of controllable and measurable quantum states known as localized spins. Localized spins that appear in NV centers can have their orientation aligned simply by shining light on them (spin initialization), indicating that these localized spins are controllable quantum states. The underlying principles of this phenomenon will be explained in detail in another article. The excellent controllability is a key characteristic of diamond NV centers. Currently, diamond NV centers are among the most advanced in terms of achieving highly accurate initialization of localized spins.
Regarding measurability, the state of localized spins can be read out using a technique called Optically Detected Magnetic Resonance (ODMR), which allows the spin state to be read out using light. Diamond NV centers fulfill the first condition that quantum sensors should meet.
Furthermore, localized spins can be thought of as tiny magnets. When these localized spins are exposed to an external magnetic field, they are influenced by that magnetic field, causing a change in their orientation (more precisely, it induces a phenomenon known as Zeeman splitting in university-level education). In this way, localized spins are affected by external magnetic fields, fulfilling the second condition that quantum sensors should meet.
You might wonder, "Why bother measuring magnetic fields?" However, as mentioned in the previous explanatory article, magnetic field measurements are crucial in various applications such as magnetoencephalography (MEG) and monitoring the capacity of car batteries. Another significant feature of diamond NV centers is that this phenomenon can be observed at room temperature. So, have you understood why researchers worldwide are paying so much attention to the "defects" in diamonds now?